Lake Washington Story
Watershed overview
Lake Washington is the largest of the three major lakes in King County, and the second-largest natural lake in the State of Washington. Lake Washington has two major influent streams:
- The Cedar River, at the lake’s southern end, contributes about 57% of the annual hydraulic load and 25% of the phosphorus load.
- The Sammamish River, at the northern end, contributes 27% of the hydraulic load and 41% of the phosphorus load.
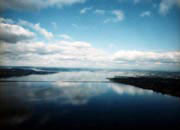
The majority of the immediate watershed is highly developed and urban in nature, with 63% fully developed. The upper portion of the watershed is the headwaters of the Cedar River that lie in the closed Seattle Water Department watershed.
The basin of Lake Washington is a deep, narrow glacial trough with steeply sloping sides. It was sculped by the Vashon ice sheet, the last continental glacier to move through the Seattle area.
The lake is 20.6 feet above mean lower tide in Puget Sound, to which it connects via Lake Union and the Lake Washington Ship Canal. The Ship Canal, constructed in 1916, is the only discharge from lakes Sammamish and Washington via the locks and dam at the western end.
Before construction of the canal, the only significant inflow was from the Sammamish River in the north. Construction of the canal resulted in the lowering of the lake 9 feet to its present level, leaving the Black River dry and the Cedar River diverted into Lake Washington.
Mercer Island lies in the southern half of the lake, separated from the east shore by a relatively shallow and narrow channel, and from the west shore by a much wider and deeper channel. In comparison to Lake Sammamish, Lake Washington is about twice as deep, four times the area, and flushes about as frequently.
Factors influencing water quality
Human activities have a major influence on water quality, but other seemingly subtle biological activities also have great significance. Lake Washington is an interesting example of how human influences and biological processes can alter water quality.
Between 1941 and 1963, the lake received increasing amounts of secondary treated sewage. This resulted in eutrophication and declined water quality of the lake.
In 1955, oceanographer George Anderson discovered the cyanobacteria Oscillatoria rubescens (formerly called a blue-green alga) in the lake. Further research suggested that nutrient conditions would soon be stimulating nuisance algal conditions, as had been documented in Lake Zurich in Switzerland.
Unlike algae, which assails the eyes and the nose, some factors of water quality are invisible and can be measured only in the laboratory. Dr. W.T. Edmondson, former professor of zoology at the University of Washington, studied the biology and chemistry of Lake Washington for many decades. Dr. Edmondson's studies implicated phosphorus from sewage as being the element from treatment-plant effluent that fertilized algae in Lake Washington.
Phosphorus was found in concentrations of 70 parts per billion in the 1960s. That was enough to feed the significant growth of algae that darkened the water and washed ashore to rot and smell. This finding had major implications for industry, and great political discussion resulted.
Lake restoration and the West Point sewage treatment plant
Lake Washington is perhaps the best example in the world of successful lake restoration by the diversion of sewage. The lake’s restoration has been extensively studied and researched.
The Municipality of Metropolitan Seattle, or Metro, was established in 1958 and given the task of diverting sewage from the lake. Between 1963 and 1968, more than 100 miles of large trunk lines and interceptors were installed to carry sewage to treatment plants built at West Point and Renton. Treated effluent ended up in Puget Sound, where currents and tidal actions diluted it.
At the time, the $140 million campaign was considered the most costly pollution control effort in the country. The investment paid off: just as Dr. Edmondson had predicted, completing these major facilities brought dramatic results. Effluent, which was at one time entering Lake Washington at the rate of 20 million gallons per day, was reduced to zero discharge in February 1968. After the last lakeshore treatment plant was closed, the concentration of phosphorus dropped quickly to about 16 parts per billion, a level maintained into the 1990s and beyond.
Blue-green algae decreased to insignificant levels. The lake's transparency, as low as 30 inches in 1964, reached 10 feet in 1968. Water quality would continue to improve: in later years the transparency would reach depths of 17 to 20 feet, with a maximum depth of nearly 25 feet in 1993.
Changes to algae, zooplankton, and fish
The improvements in water transparency went beyond what could be expected from the measured amount of phosphorus. Changes in the populations of algae, zooplankton, and fish were also a factor. Four species in particular played key roles.
Oscillatoria: thriving on phosphorus
During Lake Washington’s period of eutrophication in the 1960s, the cyanobacteria Oscillatoria rubescens was a prominent nuisance, forming thick masses near the surface of the water. This species is relatively long and filamentous, generally unsuitable food for grazing zooplankton. Oscillatoria also inhibits other algae through the physical impact of shading and through biochemical means.
Since phosphorus is a necessary nutrient for Oscillatoria, the species thrived in the phosphate-rich lake water. With the sewage diversion from the lake and resulting decrease in available phosphorus, conditions were no longer ideal for Oscillatoria, and it diminished entirely in 1976.
Daphnia: an algae-eating crustacean
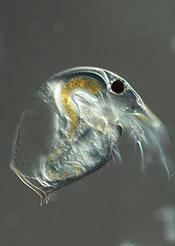
Daphnia, commonly called a water flea, is a filter feeding planktonic crustacean about 2 mm long. It suddenly became an important member of the zooplankton in Lake Washington in 1976, although it had been present in small numbers previously.
Daphnia is an efficient filter feeder and can reduce algae populations quickly, thus increasing water transparency. While Daphnia can consume some kinds of filamentous plankton, Oscillatoria clogs its filtering apparatus so it is unable to feed. When Oscillatoria populations were high, Daphnia numbers remained low. With the demise of Oscillatoria in the lake, the Daphnia population increased.
Since Daphnia can reproduce quickly to exploit favorable conditions, its numbers can fluctuate dramatically through the year. Peak numbers occur in May and June when temperature and sunlight trigger increased activity.
Neomysis: Daphnia's main predator
Neomysis mercedis, the possum shrimp, is a planktonic crustacean that can reach a total length of about 14 mm (0.5 inch). This native species has been present in Lake Washington for many decades, but has been scarce since 1968.
In the late 1970s, Paul Murtaugh of the University of Washington studied the gut contents of Neomysis and concluded that it was an especially potent predator on Daphnia. Thus as Neomycin numbers decreased, the Daphnia population grew.
Longfin smelt: reducing the Neomysis population
The longfin smelt is a small anadromous fish found on the Pacific coast from northern California to northern British Columbia. There are two landlocked populations of this species: one in Harrison Lake, BC, and the other in Lake Washington. They were first observed in Lake Washington in 1960 by Robert Dryfoos, a UW student.
Studies from the UW and Washington Department of Fisheries have shown the longfin smelt to be highly selective in feeding on Neomysis. Some two-year olds were found to have a diet composed of 96% Neomysis. Younger longfin smelt are less specialized, eating Neomysis, Daphnia, and other crustacean zooplankton.
Reasons for the increase in these smelt are not obvious. The increase may be linked to improvements in breeding habitat in the Cedar River, where the vast majority of spawning takes place. Government agencies have been working to sustain salmon habitat and to control flood damage in the area, and the smelt may have benefited from these habitat improvements.
Other affected species in the lake
Sockeye salmon
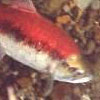
Sockeye are another species whose numbers increased during the lake's period of eutrophication, although the increase was probably not directly related to the level of phosphorus in the lake.
Fluctuating population
Sockeye are unique among salmon in that the smolts have a yearlong phase in freshwater lakes before their migration to the sea. Sockeye had been planted in the Cedar River in 1953 but were present in relatively low numbers until the mid 1960s. By 1970 the fish were numerous enough for the state to permit commercial fishing in the lake.
The increase in sockeye may have been due to some inadvertent improvements to their spawning beds. Flood control measures, and a halt to channel dredging (because of equipment failure) resulted in less silt accumulation on the gravel spawning beds.
The smolts from Lake Washington are the largest of their species, but numbers have been down in recent years. Several agencies are researching the cause of the decline. Potential causes include food supply, predation, and physical damage from the Government Locks during out-migration.
Sockeye predators
Sockeye predators include cutthroat and rainbow trout. Contrary to common notion, UW research has not shown northern squawfish to be preying substantially on sockeye in Lake Washington. The northern squawfish are adaptable in their diet, though, readily able to shift to different prey items, and could become a more active predator on the sockeye.
Largemouth and smallmouth bass are also potential sockeye predators, and one theory is that an increase in the number of boat docks has resulted in an increase in habitat for the bass. However, the spatial overlap between them and the sockeye may not be sufficient for there to be much of an impact.
Sockeye food supply
The major food supply for sockeye fry in winter is unknown. They do feed on Daphnia, but the sockeye fry appear in the lake about March or April, a month or two before Daphnia becomes abundant.
Diaptomus and Epischura
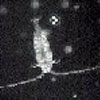
Diaptomus
Diaptomus and Epischura are genera of calanoid copepods, present in the lake year-round. The population peaks occur in early spring (March and April) and again in late summer (August and September). In contrast, Daphnia peak abundances are generally in May and June.
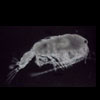
Epischura
The role of the copepods in the food chain of Lake Washington is now being investigated, because of recent declines in sockeye fry and smolts. Before the increase of Daphnia in the mid 1970s, Epischura and a summer cladoceran, Diaphanosoma, had been the preferred prey of planktivorous fish in the lake.